汽车驱动桥毕业设计-轻型货车驱动桥设计(全套含CAD图纸、三维模型)
收藏
资源目录
压缩包内文档预览:(预览前20页/共26页)
编号:12668904
类型:共享资源
大小:26.25MB
格式:ZIP
上传时间:2019-01-05
上传人:机****料
认证信息
个人认证
高**(实名认证)
河南
IP属地:河南
100
积分
- 关 键 词:
-
汽车
驱动
毕业设计
轻型
货车
设计
全套
cad
图纸
三维
模型
- 资源描述:
-






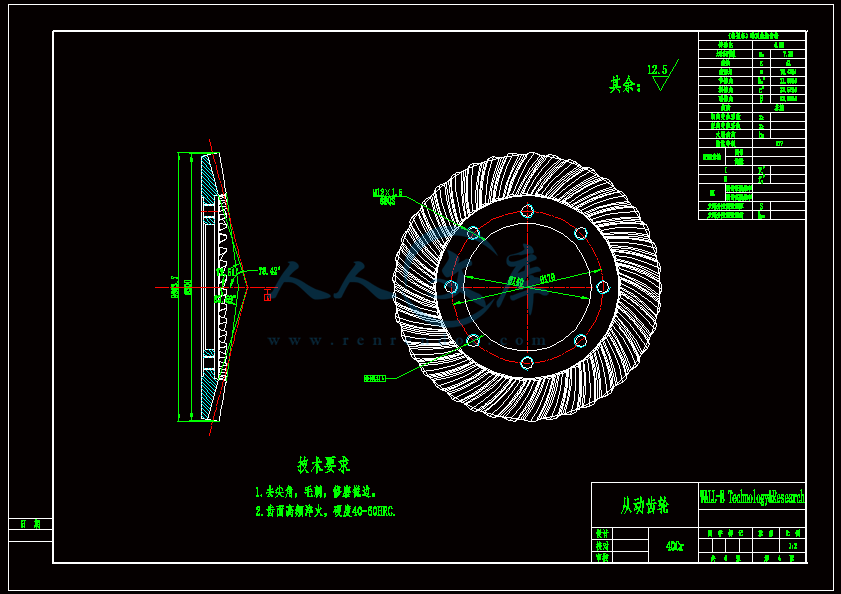





- 内容简介:
-
附 录附录A 英文文献DEFLECTION TEST AND TRANSMISSION ERROR MEASUREMENT TO IDENTIFY HYPOID GEAR WHINE NOISEJ. H. YOON1), B. J. CHOI1), I. H. YANG1) and J. E. OH2)*1)Graduate School of Mechanical Engineering, Hanyang University, Seoul 133-791, Korea2)School of Mechanical Engineering, Hanyang University, Seoul 133-791, Korea(Received 5 March 2009; Revised 13 September 2010) ABSTRACTHypoid gears are commonly used in rear-drive and 4WD (4 Wheel Drive) vehicle axles. Investigating their sensitivity to deflections is one of the most important aspects of their design and optimization procedures. Therefore, a deflection test was performed in this study in the actual gear mounting using completely processed gear. This test covered the full operating range of gear loads from “no load” to “peak load”. Under peak load, the contact pattern extended to the tooth boundaries without showing a concentration of the contact pattern at any point on the tooth surface. The transmission error was tested under an axle assembly triaxial-real-car-load condition.KEY WORDS : Hypoid gear, Deflection test, Transmission error, Gear whine noise, Axle1. INTRODUCTIONThe axle in vehicle driving systems was used primarily in small trucks, special vehicles, or buses, when FF (Front-engine Front-drive) vehicles were popular. However, as the FR (Front-engine Rear-drive), 4WD (4 Wheel Drive), or AWD (All Wheel Drive) type driving systems have been adopted in RV and SUV vehicles, which have gradually become more common since 2000, and in middle-sized or large luxury vehicles, the performance of the axle has constantly improved. As such, the importance and role of the axle continues to increase. In fact, in vehicles with improved performance, the overall indoor noise was also greatly reduced due to the use of low-noise technology, unlike in existing diesel vehicles (Lee et al., 2007; Park etal., 2008; Sim et al. , 2006). However, the gear noise in the transmission and axle, which had been masked, is increasing and has become a significant challenge. To develop a low-noise axle, the transmission error due to the input torque variation in hypoid gear must be measured; in particular, the engineers who modify the teeth to develop low-noise axles need the transmission errors of the gears in direct relation to the tooth geometry. There has been no report, however, of studies in Korea that aim to measure the transmission errors in axles. In Japan, case studies on trans-mission error measurements (Kato, 2003) were reported, but the measurement precision was imperfect. The current method of measuring torque variation is presently under examination. There have also been theories about and experimental evaluation of deflection (Coleman, 1975; Kolivand et al., 2006), but the development of the toothgeometry through the application of such theories is insufficient. Therefore, in this study, the causes of hypoid gear whine noise were identified by measuring the transmission errors under load variation and by developing experimental methods to measure the triaxle transmission errors. The results of the deflection test with the tooth geometry development of the hypoid gears that were used in the axles were then compared with the interpretation results and tests that were conducted using Gleasons T9002. DEFLECTION TEST2.1. IntroductionTo design axles, the deflection of the driving and the driven gears and the tooth contact pattern under the torque were measured. Figure 1 shows a picture of the experiment in which the deflection was measured. The tooth contact pattern was measured by applying an appropriate volume of a gear-marking compound to the gears before the two sets of gear teeth were engaged with each other to turn and by copying the imprint on the gears with tape after turning. The tooth contact pattern was then checked. Figure 2 shows the measured tooth contact pattern. Figure 1. Deflection measurement check. Figure 2. Tooth contact check.2.2. Positions of the Deflection Measurement and the Axle Noise Section of Input Torque Conditions on the Vehicle The Hypoid gear rotation direction and torque direction are shown in Figure 3. The measurement position in the direction test is shown in Figure 4, and the axes (E, P, G, and ) of the hypoid gears are shown in Figure13. Except for the upper/lower and right/left torsions of the pinion and ring gears and the displacement, the real deflections were measured with a trigonometric function (Coleman, 1975). This test result also included tooth deflection. Moreover, each displacement sensor was fixed and measured based on the inner bearing assembly of the pinion gear. Because the input revolution in the direction test was 510 rpm and because oil was not used, the test was performed with the low revolution to prevent the teeth from being damaged by the heat generated in the surface of the gear under the torque condition.Figure 3. Hypoid gear rotation direction and torque direction.Figure 4. Displacement indicator measuring point and indicator anchorage point.The noise section and the actual input torque that were measured under various driving conditions (W.O.T.: Wide Open Throttle; S.W.O.T.: Semi-Wide Open Throttle; Coast: Full Close Throttle Deceleration).Figure 5 shows a diagram of a device that measures the input torque in the axles of vehicles. Figure 6 is a graph that shows the input torque measurement for S.W.O.T. and coast driving conditions. Table 1 shows the noise section of the input torque values under coast driving conditions. It can be seen that the input torque in the gear whine noise section is between 22.5 and 7.2 kgm in real vehicles. To develop the tooth geometry with minimum transmission error under these input torque conditions, the transmission error under such input torque must be measured.Figure 5. Measurement setup of the axle input for the vehicle torque.Table 1. Axle input torque by test mode.Figure 6. Axle input torque of S.W.O.T. (semi-wide open throttle) and coast test modes.3. TRANSMISSION ERROR3.1. Transmission Error MeasurementThe general way to measure the transmission error of the hypoid gear in axles and of the helical gear in the transmission gear is to conduct the single flank test (). In this test, Gleasons 600HTT equipment is used to measure the transmission error of the gear pair. The experimental equipment is shown in Figure 7.This equipment has the following limitation: its use in the single-flank test requires that the test be performed under a low input torque/revolution speed (20 Nm /60 rpm). It can, however, check the quality of the manufactured gear pair very effectively at the manufacturing site.Figure 8 shows how the results of the experiment were analyzed. If the pinion to be measured revolves while engaged with the gears, the rotational angular velocity of each gear should be measured using a rotation angle meter whose resolution power is at least 30 times the number of gear teeth. After calculating the long and short wavesseparately according to the numbers of the pinion and gear teeth (Figure 22), the transmission error of the average tooth mesh is computed to derive the resultant value. In addition, the transmission error for each gear order can be identified by calculating each spectrum for each set of time data through the FFT process. Figure 7. Experimental setup for the single-flank measurement of the hypoid gearFigure 8. Transmission error measurement system: Gleason single-flank tester3.2. Triaxial Transmission Error MeasureThe single flank test discussed in Section 3.1 was conduced to evaluate the biaxial transmission error of the two gears. This test is inappropriate to determine the cause analysis of hypoid gear whine noise for the following reasons. First, the torque condition is low, and the deflection under the torque is not applied due to the structural limitations of the equipment. In fact, it is impossible to evaluate the transmission error under high torque because the equipment being tested is fixed to each axis by frictional jigs, not by bolts. Second, it is impossible to identify the cause of the gear whine noise because the deflection under the input torque is not applied.Figure 9 shows the engagement error meter that utilizes the revolution angle measurement. The transmission error is calculated from the revolution data of the revolution angle meter installed on the extension line of the pinion and gear axes. To prevent motor-side disturbance, the driving method with couplings and belts is utilized, but the measured value tends to change due to the revolutionary vibration of the transmission error. Figure 9. Conventional transmission error tester Figure 10. Transmission error measurements by angular velocity using laser sensors. To make up for this disadvantage, a method of measuring the transmission error using laser sensors was suggested, as shown in Figure 10. This transmission error measurement method stabilized the revolution of the axle input by using a flywheel, and it calculated the transmission error by measuring the angular-velocity variation with the use of laser sensors. This method, however, has the following disadvantages: (1) there must be a transparent hole in the body of the axle so that laser sensors can be used; (2) oil cannot be used to prevent errors due to the irregular reflection of the laser angular-velocity meter. Because the two aforementioned measurement methods use two revolution angle and angular-velocity meters by applying the single-flank test method, the precision of the measurement is reduced due to the transmission errors generated by the revolution of the differential gear, which results from the differential movement of the right/left axis generated with the fixed output axis of one side. Therefore, in this study, the revolution angles of the three axes on the axle were measured to make up for the aforementioned disadvantages of the proposed method. The transmission errors in the input torque section of the axle, which has noise sections in vehicles, were measured using the triaxial transmission error evaluation. This method of evaluating the triaxial transmission error of the axle may also be applied to FF manual transmission and its axle. Figure 11 shows the processes of configuring and computing the triaxial transmission error, and Figure 12 is a diagram of the actual triaxial transmission error measurement experiments. Three ring encoders (ERM280) of HEIDENHAIN with three Rotec E- DR counter boards were used on the input, LH output, and RH output of the axle. Each encoder rotation signal was calculated with RAS software by Rotec. Because a revolutionary angle meter has an angle revolution power of 20480 per revolution, it was possible to measure the transmission error with a high precision: 200 or more times the number of gear teeth (3080) to be measured.Figure11. Hardware and software schematic diagram of the triaxial-transmission-error tester.Figure 12. Triaxial-transmission-error measurements using a rotary encoder.4. DEFLECTION UNDER THE INPUT TORQUE4.1. Deflection under Input Torque of the AutomobileFigure 13 shows the coordinate system of the hypoid gear. Figure 14 shows the results of the deflection test under the axle input torque of the E (offset direction), P (piniondirection), and G (gear direction) axis, and Figure 15 shows the angle. Table 2 shows the result of the gear tooth contact test under the input torque. The variation of the tooth contact shows spreads out toward the heel as the torque increases in the typical Toe Bias In pattern. According to the input torque, the P axis tends to show a +direction on the drive side of the gear contact tooth when accelerating a vehicle, and a direction on the coast side of the contact gear when decelerating a vehicle. The generated deflection was between 0.02 mm and 0.025 mm. In the case of the G axis, both the accelerating tooth (drive side) and the decelerating tooth (coast side) were deflected in the same direction, and the deflection was 0.042 mm when decelerating (coast) and 0.018 mm when accelerating, which means that deflection is two times more sensitive when decelerating (coast). For the E axis, the deflection was 0.03 mm when accelerating (drive), which is lower than 0.045 mm when decelerating. For the axis, it was 89.96 when decelerating (drive), which means thedeflections are more sensitive than when accelerating (drive). Figure 13 Axial coordinate of the hypoid gear. Figure 14 EPG axial deflection test results. Figure 15. -axial deflection test result. Figure 16. Triaxial rpm measurement results in the time domain.4.2. Triaxial Transmission Error Measurement ResultsEleven axle pinion gears and 43 gear teeth were used in the test. As in Figure 11, the results of the triaxial transmission error measurement shown in Figure 16 show the measured revolution number of the time axis of the three channels (input, LH output, RH output) in the hardware. The average rpm of the two output axis rpms that were measured is shown in Figure 17. The average rpm of the two output axes was calculated because the ratio of the output axis rpm to the input rpm is always stable mechanically because the output axis is connected to the differential gear at the center. Therefore, when measuring the transmission error of the three axes of the axle, the average rpm of the gear can be calculated by computing the average of the two rpms because there is a difference between LH and RH. Figure 18 shows the angular displacement over time. This wave form resulted from the measurement of each dis-placement against the pinion gear with the revolution of the pinion gear, i.e., it is not the averaged result. Figure 19 shows that the short wave passed through the high passfilter and the long wave through the low pass filter in the total signal. Here the long wave represents the runout volume of the pinion gear, and the short wave represents the transmission error of the pinion gear. Like Figure 19, Figure 20 shows the transmission error of the gear. Figure 21, on the other hand, shows the averaged transmission error of the tooth pitch when the pinion and gears rotate while engaged with each other. Generally, the transmission error was analyzed against the 1st = A1, 2nd = A2, and 3rd = A3 orders, which corresponded with the mash order of the pinion, by analyzing the result of the FFT shown in Figure 21. The values of each order were the representative values of the transmission errors of the pinion and gears.Table 2. Tooth contact pattern results. Figure 17. LH and RH output shaft rpm averaging results in the time domain. Figure 18. Total signal by reference input Figure 19. Total signal and long and shortpinion gear rpm signal. waves of the pinion gear. Figure 20. Total signal and long and short Figure 21. Average tooth pitch result.waves of the gear.5. DESCRIPTION OF THE DEVELOPMENT OF COAST NOISE BY DEFLECTION DATA5.1. Coast Noise DevelopmentTo identify the cause of the coast noise that occurs during driving, the transmission er- ror due to input load was measured. Figure 22 shows the transmission error measure-ment results. The major cause for coast noise was the high transmission error of 14 rad or above in the zone of the torque that works as the input torque to the axle as the vehicle decelerates. The rectangular dotted line indicates the torque zone that is associated with the axle input. To reduce the noise that occurs during coasting, as shown in Figure 23, the transmission errors for each input torque were compared with a 0.10.15 mm lengthened pattern on the coast side. The results from the deflection test of E, P, G, a n d were applied using the S/W of Gleason Hypoid gear. In Figure 24, the calculation results of the transmission error for each input load before and after the change in the length of the coast side pattern were compared. The gear was produced by verifying the corrected gear teeth on the coast side with the calculation results that the transmission error value is lowered in the torque zone where the noise occurs as the vehicle coasts. Figure 25 shows the results from the comparison between the gear teeth of the coast noise gear and long contact pattern gear. In the figure, the top plot compares results from the coast side noise gear and long contact pattern gear on the drive side, while the bottom compares results on the coast side. The points that show the difference between two gear teeth are indicated with a rectangular dotted line. From point 3 to point 9 in the heel direction, the teeth value is relatively negative () and continues to be lowered in the heel direction. The axle was assembled with long contact pattern gear, and the transmission error at axle assy was measured. Figure 26 compares the transmission error results from the coast noise gear axle assy and the long contact pattern gear axle assy across the input torque. The rectangular dotted line indicates the zone to which the axle input torque is applied when the vehicle decelerates. It is consistent with the transmission error calculation results in Figure 24. Figure 27 shows the measurement results of interior noise when the vehicle decelerates. In the zones from 80 to 90 kph, 95 to 110 kph and 120 to 130 kph, where coast noise was problematic, the noise level was reduced by 58 dB (A). Figure 22. Transmission error of coast Figure 23. Development method of coastnoise axle test result. noise hypoid gear. Figure 24. Transmission error calculation res- Figure 25. Comparison of coastult of coast noise gear and long contact pattern. noise gear and long contact patterntooth profile measurement results. Figure 26. Transmission error measurement Figure 27. Car test results of coast noiseresults of the coast noise axle and long co- axle and long contact pattern axle #1 andntact pattern axle. #2.6. CONCLUSIONTo identify the gear whine noise in the axle composed of a hypoid gear and reduce the noise in a decelerating vehicle, the corrected gear teeth and transmission error measures were compared by applying the amount of deflection in the input torque zone of the noise occurring zones. The gear produced based on these results also confirmed that the transmission error from input torque shows the same trend in axle assy. In addition, the noise level was reduced by 58 dB (A) in the actual vehicle in the noise-occurring zone. These findings suggest that the occurrence of hypoid gear whine noise will be minimized if, based on these results, the target range of the axle input torque for the development of optimal hypoid gear is measured and set as the range of axle input torque and if the amount of transmission error from input torque is verified analytically for corrected teeth gear; moreover, the consequences of applying the deflection measurement results and reflected onto the product are determined.REFERENCESColeman, W. (1975). Analysis of mounting deflections on bevel and hypoid gears. SAE Paper No. 750152.Kato, N. (2003). Measuring method of transmission error of final reduction gearboxes and gear noise occurrence mechanism. JSME, 69 , 230 235.Kolivand, M., Hannaneh, A. and Soltani, N. (2006). Retaining hypoid gear performance characteristics with differ- ential housing and shafts deflections. ASME, IMechE 2006-13216, 361 369.Lee, Y. Y., Park, S. G. and Oh, J. E. (2007). A study on vibration transfer path identification of vehicle drivers position by multi-dimensional spectral analysis. Trans.Korean Society for Noise and Vibration Engineering, 17 , 741746.Park, S. G., Park, W. S., Sim, H. J., Lee, J. Y. and Oh, J. E. (2008). Sound quality evaluation and grade construction of the level D noise for the vehicle using MTS. Trans.Korean Society for Noise and Vibration Engineering, 18 , 393399.Sim, H. J., Ryu, J. S., Cha, K. J. and Oh, J. E. (2006). The optimal design for noise reduction of the intake system in automobile using Kriging model. Trans. KSME(A) ,30 , 465 472.附录B 中文翻译挠度试验和传动误差测量识别准双曲面齿轮噪声1.机械工程研究所、汉阳大学133 - 791年首尔,韩国2.机械工程学院、汉阳大学133 - 791年首尔,韩国(2009年3月5日; 2010年9月13日修订)摘要 准双曲面齿轮常用在后驱车和全驱车上,挠度的灵敏度的研究对车的设计和优化起到至关重要的作用,因此挠度试验在实际齿轮安装过程中是必不可少的。此试验涵盖了汽车从空载到满载的所有工作情况,在满载时齿轮接触区域延伸到齿轮的全部齿面,传动误差试验是以三轴总成的车为载体进行的。关键词: 准双曲面齿轮、挠度试验、传动误差、齿轮噪声、轴1. 介绍当前置前驱的车很受欢迎时,行驶系统中的驱动桥主要用于小卡车,特种车或公交车等。然而,随着前置后驱,四驱和全轮驱动式驱动系统在自2000年以来越来越常见的房车和运动型多用途车等中或大型豪华车上的应用越来越广时,轴的性能和重要性也在不断提高。事实上,由于采用了低噪音技术,与柴油车辆相比,在车辆性能上明显提高,整体室内噪音也大大减少了,然而在传动系统和驱动桥的噪声经常被忽略,它也成为了一个重大挑战。为了开发一个低噪音的轴,由于准双曲面齿轮输入转矩的变化,应测量传动误差,特别是工程师研发低噪声的驱动桥需要测量直接关系到齿的几何形状的齿轮传动误差,然而在韩国还没有关于轴上齿轮传动误差的研究性报告。在日本曾经有过关于传动误差测量的研究,但是其测量精度却不令人满意。目前测量转矩变化的方法还在考察之中,现在也有关于传动误差的理论和实践评价,但是通过这样的理论和实践评价来确定发展齿牙的几何学是不充足的。因此在这项研究中,在负荷变化和测量实验的传动误差试验中能够证明双曲面齿轮噪声大的原因。在轴上的双曲面齿轮挠度试验和齿轮啮合传动误差的结果之后和T900格里森公司的结果进行比较。2. 挠度试验2.1 介绍设计轴时,应测量在运动时驱动桥,驱动齿轮的挠度和齿牙的接触形式。图1显示了挠度试验是怎样进行的,其通过测定齿面接触区由施加适当量的复合齿轮的两个系列齿轮的轮齿与另一个进行转变和复制,之后再测量齿轮接触形式。图2显示了齿轮的接触形式。 图1 挠度试验测量 图2 齿轮接触形式2.2 挠度试验的位置和车轮运动时驱动桥噪音产生区域图3显示了准双曲面齿轮的旋转方向和输入转矩的方向,图4显示了方向试验的测量位置,图13显示了准双曲面齿轮的轴线方向。除了大小齿轮的扭矩和位移之外,实际挠度是由一个三角稳定装置测量的。实验结果也包括齿牙挠度的测量。此外,每个位移传感器的固定和测量是以小齿轮内轴承的安装为基础的。为了防止在未使用润滑油的情况下高速旋转使齿轮破坏,在测量方向上的输入转矩速度为每分钟510转。噪音区域和实际输入转矩应在不同的工作环境下测量。 图3 准双曲面齿轮旋转方向和扭矩传递方向 图4 位移测量点指示器和传递点指示器图5展示了测量驱动桥输入转矩的装置图,图6展示了在半抱死和全抱死状态下输入转矩的变化图表,表1展示了在全抱死状态下输入转矩的噪声区域。实验表明在22.5 7.2 kgm转矩范围内噪声明显,为了研究在这样的输入转矩条件下齿轮传动的最小传动误差,此时的传动误差需要测量。 图5 汽车驱动桥输入转矩的测量装置表1 驱动桥输入转矩测量数据 图6 在半抱死和全抱死状态下的输入转矩3. 传动误差一般方式来测量准双曲面齿轮和斜齿轮的传动误差是在单侧面进行测试,在这次实验中600 HTT格里森公司的设备被用来测量齿轮副传动误差。图7显示了试验的设备,该设备具有以下的局限性: 在做单侧面试验时应在低输入转矩或低转速条件下进行(20 Nm /60 rpm),然而在加工现场却能非常有效地检查齿轮副的质量。图8展示了如何分析实验的结果。如果被测齿轮正在旋转,齿轮转动角速度应使用旋转角测量仪测量,测量仪的转动速率至少是轮齿的三十倍。在根据大小齿轮的啮合数量计算长波短波后,传动误差是啮合齿传动的平均误差。此外,通过FFT的过程,每个齿轮的传动误差可以由一系列的时间数据光谱计算出来。 图7 双曲面齿轮单侧面测量仪器 图8 传动误差测量系统:格里森单侧面测量仪3.2 三轴的传动误差测量在3.1时我们讨论的是双轴的传动误差单侧面测量试验,但用这个试验来分析双曲面产生噪声的原因是不恰当的,理由如下:首先,转矩小,在此转矩下产生的挠度没有达到材料结构的极限值,而实际上在高转矩下的产生的传动误差是不能测量的,因为被测量的设备上的轴是由摩擦力固定的而不是用螺栓固定的。其次,它不能测定齿轮产生噪声的原因是在此转矩下的挠度不适用。图9展示了旋转角度测量仪,传动误差即是由安装在齿轮渐开线处的测量仪的数据测算出来的。为了避免汽车运动产生干扰,驾驶时应注意离合器和传动系的配合,但传动误差的值还是会随着汽车的振动而改变。 图9 传统的传动误差测量仪图10 利用激光传感器测量角速度的传动误差测量装置为了弥补这些不足,利用激光传感器测量角速度的传动误差测量装置应运而生,如图10所示。使用飞轮可以使输入轴旋转的测量误差更加稳定,通过激光传感器测量角速度
- 温馨提示:
1: 本站所有资源如无特殊说明,都需要本地电脑安装OFFICE2007和PDF阅读器。图纸软件为CAD,CAXA,PROE,UG,SolidWorks等.压缩文件请下载最新的WinRAR软件解压。
2: 本站的文档不包含任何第三方提供的附件图纸等,如果需要附件,请联系上传者。文件的所有权益归上传用户所有。
3.本站RAR压缩包中若带图纸,网页内容里面会有图纸预览,若没有图纸预览就没有图纸。
4. 未经权益所有人同意不得将文件中的内容挪作商业或盈利用途。
5. 人人文库网仅提供信息存储空间,仅对用户上传内容的表现方式做保护处理,对用户上传分享的文档内容本身不做任何修改或编辑,并不能对任何下载内容负责。
6. 下载文件中如有侵权或不适当内容,请与我们联系,我们立即纠正。
7. 本站不保证下载资源的准确性、安全性和完整性, 同时也不承担用户因使用这些下载资源对自己和他人造成任何形式的伤害或损失。

人人文库网所有资源均是用户自行上传分享,仅供网友学习交流,未经上传用户书面授权,请勿作他用。